This vision study will identify and explore each of these transformative pathways and how they can be pursued together to chart a course to an industrial transformation.
Industrial Efficiency & Decarbonization Office
January 17, 2025A Framework for Industry To Meet the Next Defining Moment for American Innovation
The United States is rapidly nearing a transformation that will depend on continued American innovation. We have a once-in-a-generation opportunity to grow the industrial sector and sharpen our competitive edge and national security globally, while bending the sector’s upward greenhouse gas emissions trend.
Leveraging the opportunity before us will require a full-scale industrial transformation—fundamentally reimagining a sector that has been optimized over hundreds of years and carefully considering its impact across American communities and the globe.
Our nation can pursue many pathways in parallel across each individual subsector to bring about an industrial transformation. This vision study will identify and explore each of these transformative pathways and how they can be pursued together to chart a course to an industrial transformation.
Jump to:
To read the full report, download the PDF: Transformative Pathways for U.S. Industry: Unlocking American Innovation.
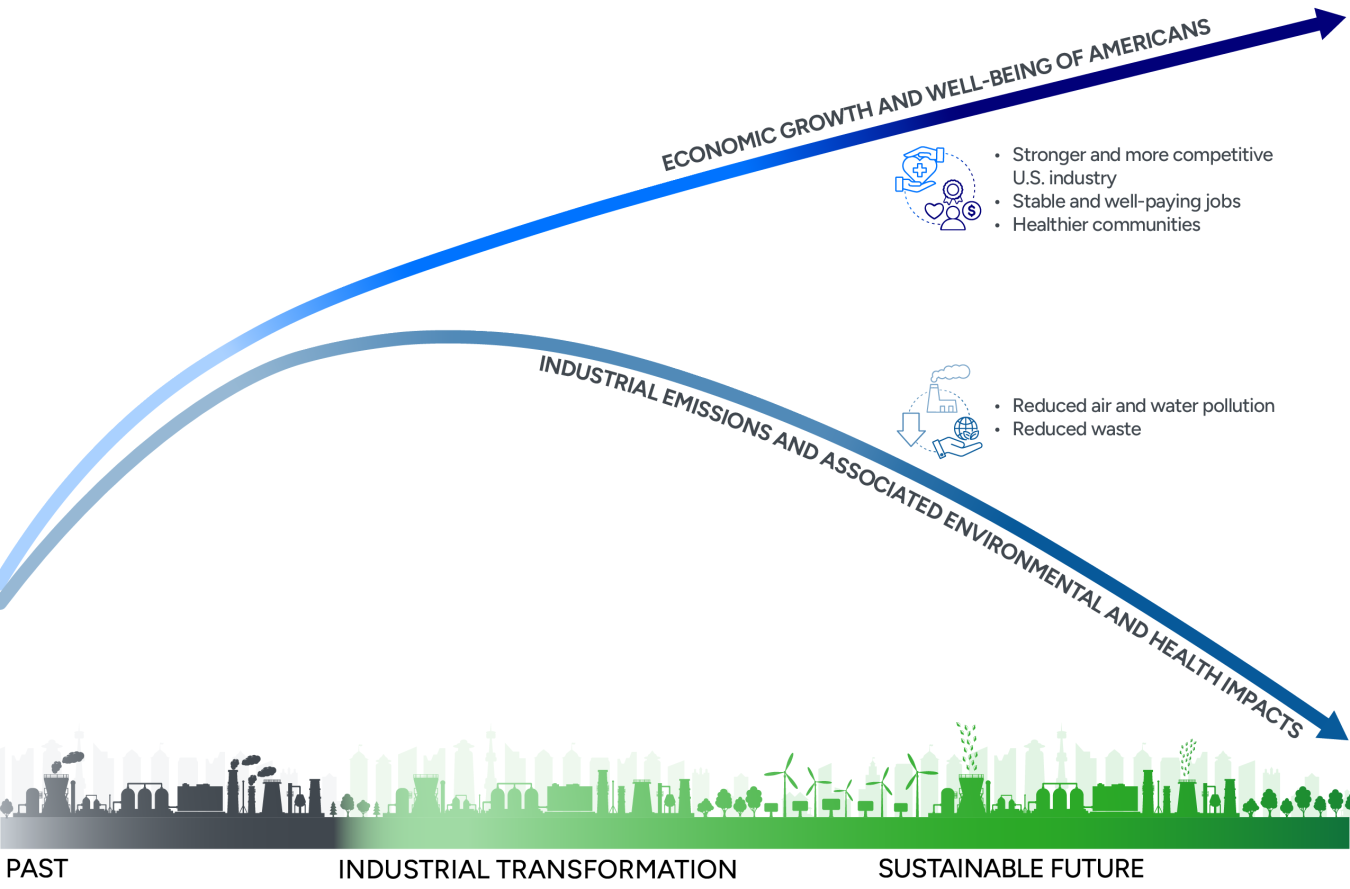
The economic growth and well-being of Americans includes healthier communities, stronger and more competitive U.S. industry, and stable and well-paying jobs. The industrial emissions and associated environmental impacts includes reduced greenhouse gas emissions, reduced criteria air pollutants, reduced water pollution, and decreased impacts on biodiversity.
The U.S. industrial sector accounts for approximately 38% of total U.S. economy emissions. Under business-as-usual (BAU) operations, the U.S. industrial sector’s energy consumption and energy-related CO2 emissions are projected to grow significantly by 2050.
To curb these emissions, we will need to quickly move state-of-the-art emissions reduction technologies to today’s factory floors while continuously innovating to develop the next wave of breakthrough ideas.
Pillars of Industrial Decarbonization
The 2022 Industrial Decarbonization Roadmap continues to provide the framework for DOE’s industrial decarbonization strategy and outlines technology opportunities and potential challenges for five major manufacturing subsectors: cement and concrete, chemicals, food and beverage, iron and steel, and petroleum refining.
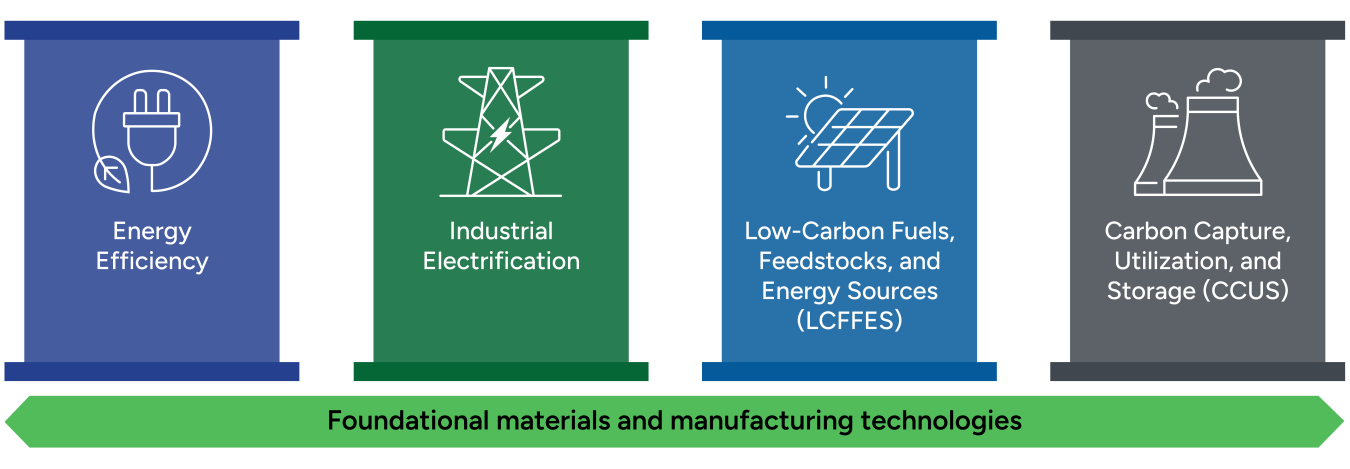
The pillars of industrial decarbonization are:
- Energy efficiency: Advancements that minimize industrial energy demand, directly reducing the greenhouse gas emissions associated with fossil fuel combustion.
- Industrial electrification: Technologies that utilize electricity for energy, rather than combusting fossil fuels directly, enable the subsector to leverage advancements in low-carbon electricity from both grid and onsite generation sources.
- Low-carbon fuels, feedstocks, and energy sources (LCFFES): Substitutions for fossil-based fuels, feedstocks, and energy sources to further reduce combustion- and process-associated industrial emissions.
- Carbon capture, utilization, and storage (CCUS): Multicomponent strategy for mitigating difficult-to-abate emissions, involves capturing generated CO2 before it can enter the atmosphere; utilizing captured CO2 whenever possible; and storing captured CO2 long-term to avoid atmospheric release.
In addition to the roadmap, this vision study builds upon DOE’s Pathways to Commercial Liftoff reports, which focus on commercial considerations for near-term technology adoption. The Liftoff reports utilized extensive stakeholder engagement and the best available cost estimates to provide a fact base to public- and private-sector capital allocators. These reports offer a perspective as to how and when various technologies could reach full-scale commercial adoption.
Frameworks for Informed Decision Making
A transformative decarbonization pathway is a sequence of technology deployments and retirements over time that allow U.S. industry to arrive at an established level of greenhouse gas emissions in an established timeframe.
Transformative pathways are not a single decision, but rather a series of decisions over time. Transformative pathways require decision making and investment under uncertainty. All transformative pathways require parallel investments to achieve net zero greenhouse gas emissions by 2050. Due to the long lifetimes of industrial facilities and related infrastructure, timing is challenging for any pathway.
We have developed data-informed decision tree frameworks to visualize the optionality to help stakeholders evaluate and understand potential transformative pathways. These frameworks represent a continuous process that can be applied at different points of time.
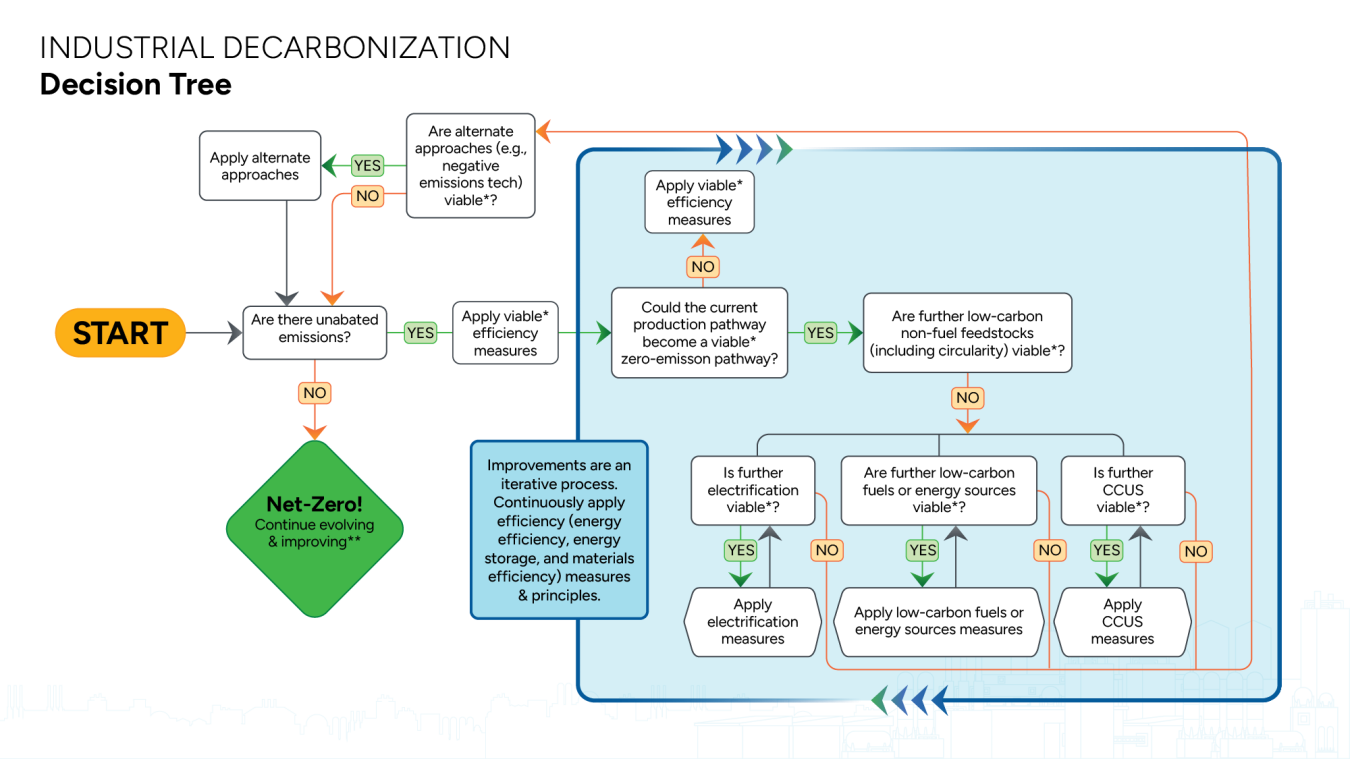
* Viable implied currently available, cost-effective, and that the measures are deemed effective through societal and environmental criteria and necessary
** Morrow, William III et al. 2017, “U.S. Industrial Sector Energy Productivity Improvement Pathways.” https://www.aceee.org/files/proceedings/2017/data/polopoly_fs/1.3687847.1501159031!/fileserver/file/790251/filename/0036_0053_000067.pdf.
Cement and Concrete
Concrete, of which cement is the primary component, is the second most used substance in the world after water and is essential in building our nation’s infrastructure. The U.S. cement and concrete subsector comprises a mix of a few multinational companies that collectively own a majority of installed cement capacity and several small and medium enterprises. The subsector accounted for $37 billion in value added and employed over 176,000 workers in 2021. Cement production in the United States is relatively dispersed throughout the country at 96 plants across 34 states and Puerto Rico, based on 2018 data. Emissions from the cement subsector can be attributed to both process emissions and energy use. Transforming the cement and concrete subsector to reach near zero emissions will require major shifts in the way cement and concrete are produced and used.
- The two near zero transformative pathways have the potential to reduce the subsector’s emissions to approximately 10 MMT CO2e, an 85% reduction compared with 2018:
- High Clean Clinker Production, Moderate SCM
- Moderate Clean Clinker Production, High SCM.
- The transformative pathways were not fully distinct in that they both increased usage of SCM to differing degrees (represented as limestone calcined clay in the modeling) and clean clinker production (represented as CCS-enabled clean clinker production in the modeling).
- The modeling was limited in that it lacked implementation of novel clinker production routes, such as those using electrochemical conversion, or nascent technologies for clinker alternatives.
- Both modeled transformative pathways leveraged CCS-enabled clean clinker production to represent 80%–100% of clinker production in 2050. The transformative pathways differed in the amount of clinker demand required in 2050, as higher SCM adoption resulted in lower clinker demand. Both transformative pathways showed significant demand reduction in clinker, such that clinker production coalesced around sites more suitable for geologic storage.
- Clean clinker could alternatively be accomplished with novel clinker production routes (not modeled) separately or in combination with CCS-enabled clinker production.
- For novel clinker production routes to have any appreciable market share by 2050, they must be commercially viable against conventional clinker pyroprocessing with CCS before 2035.
- Both modeled transformative pathways leveraged SCMs to reduce clinker content in cement in 2050, 40% for the aggressive turnover pathway and 60% for aggressive SCM pathway. Utilizing SCMS to offset clinker represents a decarbonization intervention with near-term greenhouse gas reduction at the risk of locking in a feedstock with significant embodied emissions. These embodied emissions limit the long-term potential of a pathway utilizing SCMs unless these embodied emissions are also addressed, as they are in aggressive SCM pathway:
- As clinker production moves towards clean production, tradeoffs between the emissions of SCMs versus clinker as well as other environmental, economic, technological, and societal impacts need to be balanced.
- In addition to clean clinker production, the analysis also identified fuel-switching from coal and petcoke to natural gas and biomass as a decarbonization opportunity. Although the impacts on greenhouse gas emissions may be less, they are still necessary to achieve near zero greenhouse gas emission by 2050.
- The two near zero transformative pathways have the potential to reduce the subsector’s emissions to approximately 10 MMT CO2e, an 85% reduction compared with 2018:
The two plots below (click each tab) show the necessary actions needed to reach near zero emissions for the clean clinker production with aggressive SCM adoption pathway and clean clinker production with moderate SCM adoption pathway.
This graph shows U.S. annual greenhouse gas (GHG) emissions from 2018 through 2050 for the clean clinker production with aggressive supplementary cementitious materials (SCM) adoption pathway. Labels refer to:
- Moderate Increase in SCM Use: Clinker-to-cement ratio drops to 0.6, about 0.3 kilograms of limestone-calcined clay cement (LC3) per kilogram of cement, and LC3 carbon intensity (CI) reduces by 50% by 2050.
- Clean clinker production:
- Fuel Switching: Coal and petcoke phased out, natural gas = 78% and biomass = 13% of thermal input by 2050.
- High Turnover with Limited CCS: Incumbent clinker technologies = 20% market share by 2050, shift to dry kilns with carbon capture and sequestration (CCS) (mostly conventional, some fuel-based indirect heating), only half the CO2 is captured and stored, minimal calciner/kiln electrification.
- Aggressive Turnover with Unlimited CCS: Incumbent clinker technologies phased out, dry kilns with CCS = 60%, and calciner/kiln electrification = 40% of market share by 2050, 95% of CO2 is captured and stored.
- Electric Grid Decarbonization: Net zero greenhouse gas grid by 2050.
This graph shows U.S. annual greenhouse gas (GHG) emissions from 2018 through 2050 for the clean clinker production with moderate SCM adoption pathway. Labels refer to:
- High SCM Use: Clinker-to-cement ratio drops to 0.4, about 0.5 kilograms of limestone-calcined clay cement (LC3) per kilogram of cement, and LC3 carbon intensity (CI) reduces by 50% by 2050.
- Near Zero SCMs: LC3 CI reduced by 90% by 2050 through carbon capture and sequestration (CCS) and electrification.
- Clean clinker production:
- Fuel Switching: Coal and petcoke phased out, natural gas = 78% and biomass = 13% of thermal input by 2050.
- High Turnover with Limited CCS: Incumbent clinker technologies = 20% market share by 2050, shift to dry kilns with CCS (mostly conventional, some fuel-based indirect heating), only half the CO2 is captured and stored, minimal calciner/kiln electrification.
- Unlimited CCS: 95% of CO2 is captured and stored.
Chemicals
The U.S. chemicals subsector is broad and diverse. It produces over 70,000 products from more than 11,000 facilities across the United States. The subsector accounted for $472 billion in value added and employed over 750,000 workers in 2021. Complex interdependencies between different processes and the diversity of chemical production present unique challenges to decarbonization. Emissions in this subsector stem from fuel combustion, the use of sorbents and carbonates, and various industrial processes.
This analysis primarily focused on nine high-volume, energy- and emissions-intensive basic chemicals: ethylene, propylene, butadiene, benzene-toluene-xylene (BTX), chlor-alkali (co-production of chlorine and sodium hydroxide), soda ash, ethanol, methanol, and ammonia. Together, these chemicals account for roughly 40% of total U.S. chemicals subsector greenhouse gas emissions in 2018. The remaining 60% of subsector emissions come from the production of hundreds of other chemicals. This analysis considers cross-cutting measures to decarbonize these chemicals but does not evaluate process-level decarbonization pathways.
- The core near zero transformative pathway for the modeled chemicals* has the potential to reduce the emissions to approximately 15 MMT CO2e by 2050, an 84% reduction compared with 2018.
- This subsector is especially challenging to decarbonize given the wide range of products, large thermal footprint, current reliance on energy- and emissions-intensive feedstocks and processes, and the dependence on co-manufacturing of certain chemicals.
- While interventions along the decarbonization pillars provide substantial decarbonization potential, innovative clean chemicals production technologies are needed to reach near zero emissions (represented as CCS-enabled clean production in the modeling). Without these innovations, achieving near zero or absolute net zero emissions seems unfeasible for most chemicals, with the exception of ethanol and chlor-alkali.
- Transformative pathways and pillar impacts will vary by chemical; for example, ethanol has a higher CCUS emissions reduction potential compared to some of the other chemicals modeled due to large proportions of high-purity CO2 emissions.
- Methanol, ethanol, ammonia, and CO2 are critical feedstocks for future chemicals manufacturing that should be prioritized for further research.
- The production costs of clean hydrogen underpin the economics of methanol and ammonia production emissions reduction.
- Beyond the four decarbonization pillars, material recycling is another major lever for reducing chemicals subsector emissions, especially for those with existing recycling methods, such as ethylene, propylene, and BTX.
*The transformative pathways modeling included deep dives on nine key basic chemicals which account for 40% of 2018 subsector emissions–ethylene, propylene, butadiene, benzene-toluene-xylene (BTX) aromatics, chlor-alkali (coproduction of chlorine and sodium hydroxide), soda ash, ethanol, methanol, and ammonia–and estimated the impact of crosscutting decarbonization measures for the remaining chemicals in the subsector. The near zero pathway was for eight chemicals– ethylene, propylene, butadiene, BTX, chlor-alkali, soda ash, methanol, and ammonia.
The plot below shows the necessary actions needed to reach near zero emissions for the core near zero pathway.
This graph shows U.S. annual greenhouse gas (GHG) emissions for chemicals in a core near zero pathway. The labels indicate:
- Demand Reduction: Chemical demand decreases due to increased recycling and greater material efficiency.
- Technology Transition: Adoption of best available technologies, electric heating technology, and low-carbon routes (including electrified and bio-based); simultaneous grid decarbonization to zero by 2050.
- Autonomous Improvements and Fuel Switching: Automatic technology improvements ranging from 0.03% to 0.5% per annum. Although the fuel mixes in technology transition overlap with fuel switching, this refers specifically to the increased use of biofuels.
- Clean Chemicals Production: Approaches can include carbon capture and sequestration and/or emerging chemical production processes that reduce greenhouse gas emissions compared with incumbent approaches.
Food and Beverage
The food and beverage subsector is widely dispersed throughout the country with over 38,000 facilities nationwide. It accounted for $463 billion in value added and employed over 1.7 million workers in 2021. The food and beverage supply chain—from the farm to the consumer—is incredibly complex and interconnected, with various facilities producing vastly different products at vastly different scales. These challenges, combined with limited data availability across the supply chain, make determining the energy or emissions of any given product difficult. Thus, this analysis only focuses on emissions reductions from the manufacturing stage and includes six food and beverage manufacturing subsectors: grain and oilseed milling, sugar, fruit and vegetable preserving and specialty food, dairy products, animal slaughtering and processing, and beverages. These subsectors accounted for 78% of food and beverage manufacturing greenhouse gas emissions in 2018.
- Multiple feasible near zero transformative pathways exist for this subsector, largely driven by decarbonizing hot water, hot air, and steam production.
- These transformative pathways have the potential to reduce the subsector’s emissions to below 1 MMT CO2e by 2050, a 99% reduction compared with 2018.
- While each transformative pathway leans heavily into the impact of individual pillars, electrification, energy efficiency, and LCFFES will all be needed to reach near zero emissions. CCUS is not expected to make a significant impact in the subsector but might be considered for individual facilities with large point-source emissions.
- The most likely eventual transformative pathway would include a mix of energy efficiency measures, increases in electrification, and utilization of LCFFES (as available and appropriate. Decisions around technology and pathway choice will depend on multiple factors, including the accessibility and availability of low-carbon fuels, clean electricity, and other energy sources and the processes within individual facilities.
- No regrets strategies include investments in demonstration and deployment, especially since the subsector can greatly benefit from commercially available or mature technologies (e.g., heat pumps, dual-fuel process heating, or steam-generating equipment).
- Changes in consumer demand, including preferences for certain products and food loss and waste reduction, as well as food-safety regulations can affect the choices industrial entities make in decarbonizing their operations.
- Although the transformative pathways modeling focused on Scope 1 and 2 emissions for the manufacturing stage, it is important to consider the results in context with the entire food and beverage supply chain.
The plot below shows the necessary actions needed to reach near zero emissions in the core near zero pathway. Emissions reduction are categorized by end use hot water, hot air, steam, and others (including machine drive, process cooling and refrigeration, facility HVAC, and other process and nonprocess uses).
This graph shows U.S. annual greenhouse gas (GHG) emissions from 2018 through 2050 for the food and beverage industry in a core near zero transformative pathway. Fuel intensity reductions with high electrification:
- Steam: 74% steam-generating heat pumps (91%-94% adoption); 19% - boilers EE; 6% - electric boilers (6-8% adoption).
- Hot Air: 76% steam-generating heat pumps (81%-98% adoption); 14% dryers/ovens EE; 12% advanced electroheating technologies (11-17% adoption).
- Hot Water: 74% steam-generating heat pumps (91%-94% adoption); 19% boilers EE; 12% advanced electroheating technologies (11%-17% adoption).
- Other End Uses: Facility HVAC: boilers EE; steam-generating heat pumps (94% adoption); machine drive: fans, motors, etc. EE; process cooling and refrigeration: chillers EE; process integration.
This graph shows U.S. annual greenhouse gas (GHG) emissions from 2018 through 2050 for the food and beverage industry in a core near zero low-carbon fuels, feedstocks, and energy sources (LCFFES) pathway. Fuel intensity reductions with increased onsite use of low-carbon fuels:
- Steam: Fuel intensity reductions: 42% from steam-generating heat pumps (2050 adoption rate: 53%); 37% from LCFFES adoption; 18% from boilers EE.
- Hot air: Fuel intensity reductions: 45% from steam-generating heat pumps (2050 adoption rate: 53%); 41% from low-carbon fuels, feedstocks, and energy sources (LCFFES) adoption; 13% from dryers/ovens EE.
- Hot water: Fuel intensity reductions: 41% from hot water heat pumps (2050 adoption rate: 52%); 37% from LCFFES adoption; 18% from boilers EE.
- Others: Facility HVAC: boilers EE; hot water heat pumps (2050 adoption rate: 52%); Machine drive: fans, motors, etc. EE; Process cooling & refrigeration: chillers EE; process integration.
Iron and Steel
The U.S. iron and steel subsector produces goods that are critical to both infrastructure and, as a raw material, manufacturing. It accounted for $72 billion in value added and employed over 115,000 U.S. workers in 2021. Facilities were predominantly concentrated in the industrial regions of the Midwest and the Northeast but have more recently expanded in the South. Roughly 30% of domestic steel is produced in blast-furnace basic oxygen furnace (BF-BOF) integrated mills. The balance is produced by electric arc furnace (EAF) facilities, which can have a significantly lower carbon footprint than BF-BOF produced steel. The subsector must overcome significant challenges to decarbonize, including technological barriers, cost issues, raw material quality constraints, and scrap supply limitations.
- Two distinct transformative pathways emerged for iron and steel. These pathways align in the steelmaking and finishing stages but differ in the ironmaking stage. The distinct approaches to clean production in the ironmaking stage that define these pathways are:
- Integrated mills with carbon capture and sequestration (IM-CCS)
- Hydrogen-ready DRI (H2-DRI) with evolution towards hydrogen as fuel and reductant.
- The two near zero transformative pathways have the potential to reduce the subsector’s emissions to approximately 10 MMT CO2e by 2050, a 90% reduction compared with 2018.
- Electrolytically produced iron was limited in its representation in the Transformative Pathways modeling and severely constrained in its implementation. It is acknowledged that these nascent production routes can collectively represent another alternative pathway for clean production in the ironmaking stage.
- These distinct ironmaking clean production routes require disparate capacity build-out, supporting infrastructure, and broader decarbonization measures (e.g., CO2 pipelines or clean hydrogen availability), and decisions are required in the near-term (within the next three to five years) on which pathway to pursue. Utilizing scrap to the maximum extent possible is common to all transformative pathways.
- Regardless of the pathway, the EAF plays a significant role in steelmaking, even when maintaining high BF-BOF production capacity, due to its role in production processes with high scrap charge. Fully decarbonizing EAFs with respect to the process emissions from the charge carbon and the natural gas used primarily in the preheating is essential for a NZ greenhouse gas emissions reduction pathway and can account for up to 15 MMT of annual CO2e emissions.
- It is essential that finishing stage emissions are addressed in all transformative pathways. Even in an integrated mill with CCS, capturing emissions from the finishing stage may not be considered practical. These emissions can account for up to 20 MMT of annual CO2e emissions. Electrification or low-carbon fuels are two options for addressing these emissions.
- Two distinct transformative pathways emerged for iron and steel. These pathways align in the steelmaking and finishing stages but differ in the ironmaking stage. The distinct approaches to clean production in the ironmaking stage that define these pathways are:
The two plots below show the necessary actions needed to reach near zero emissions for the Integrated Mill carbon capture and sequestration (CCS) pathway and the H2-direct reduced iron (DRI) pathway.
This graph shows U.S. annual greenhouse gas (GHG) emissions from 2018 through 2050 for the iron and steel industry in the Integrated Mill carbon capture and sequestration (CCS) pathway. Labels refer to:
- Clean the grid: Net zero by 2050
- Adopt clean ironmaking technologies: Approaches include transition to carbon capture and sequestration (CCS)-integrated blast furnace and natural gas DRI, clean H2-direct reduced iron (DRI), and electrolysis processes
- Adopt clean-electric arc furnace (EAF) and clean-finishing
- Maximize use of H2 as a fuel
- Address the remaining 30% of integrated mills emissions (finishing stages).
This graph shows U.S. annual greenhouse gas (GHG) emissions from 2018 through 2050 for the iron and steel industry in an H2-direct reduced iron (DRI) pathway. Labels refer to:
- Clean the grid: Net zero by 2050
- Adopt clean ironmaking technologies: Primarily transition ironmaking to clean H2-direct reduced iron (DRI) and electrolysis processes
- Adopt clean-electric arc furnace (EAF) and clean-finishing
- Maximize use of H2 as a fuel.
Petroleum Refining
Petroleum refining plays a key role in the energy supply chain by delivering fuels for transportation and industrial applications, feedstocks to the petrochemical subsector, and other products. It accounted for $122 billion in value added and employed over 58,000 workers in 2021. The United States has the highest refining capacity in the world, with a total processing capacity of over 18 million barrels of crude oil per day across over 120 facilities. Alongside its importance in energy supply chains, petroleum refining is a critical subsector to decarbonize, not only to mitigate the volume of greenhouse gas emissions but also to maintain U.S. competitiveness in a low-carbon global economy.
- The core near zero transformative pathway has the potential to reduce the subsector’s emissions to approximately 7 MMT CO2e by 2050, a 97% reduction compared with 2018.
- While the impact is far less than demand reduction, achieving near zero emissions will require:
- Energy efficiency improvements using known technologies, especially in the near and mid-term.
- Producing liquid hydrocarbon-based fuels from a broader suite of alternative feedstocks, including woody biomass and agricultural wastes.
- Low CI hydrogen and CCS, both of which will require major infrastructure development.
- Deploying biofuels to displace demand for petroleum crude-derived fuels.
- Electrification may have a limited role in refining decarbonization, particularly due to the need for refiners to find a use for self-generated fuels, regardless of feedstock used in fuels production.
- In the long-term it is imperative to develop and deploy the supply chains for alternative feedstocks, including the sourcing of material, the cost-competitive conversion processes for sustainable materials, and the infrastructure to transport sustainable feedstocks (e.g., bio-oil) to refining operations.
- Reducing demand for petroleum crude-derived fuels is necessary to reach near zero emissions, even with maximizing the adoption of other decarbonization interventions. Demand reduction alone accounts for over half of emissions reduction compared with 2018.
- Refineries play a pivotal role in the transition toward a low-carbon future through the supply of low-carbon liquid transportation fuels. Thus, it is imperative that RD&D decisions for refining and transportation decarbonization be pursued in tandem and investments be balanced between onsite and product decarbonization.
The plot below shows the necessary actions needed to reach near zero emissions in the core near zero pathway.
This graph shows U.S. annual greenhouse gas (GHG) emissions from 2018 through 2050 for the petroleum refining industry in a core near zero pathway. The labels refer to:
- Demand Reduction: Global decline in use of liquid transportation fuels.
- Energy Efficient Technologies: Advanced heat exchangers, waste heat recovery, cogeneration, advanced furnace design, digitalization.
- Low Carbon Utilities: Decarbonized grid, purchased low-carbon-intensive hydrogen, renewable natural gas/biogas.
- Carbon Capture: Retrofit of onsite H2 steam-methane reforming plants, cogeneration, other individually large combustion sources.
- Biofuels: Renewable diesel/sustainable aviation fuel, coprocessing bio-oils.
Pulp and Paper
Pulp and paper manufacturing creates a wide variety of products, including graphic papers, newsprint, containerboard, linerboard, tissue, and specialty paper. This U.S. subsector accounted for $90 billion in value added and employed over 330,000 workers across roughly 5,300 facilities in 2021. The demand in types of pulp and paper products continue to change overtime with the decline of print media and growth in hygiene and packaging-related products. Decarbonizing this subsector will be largely dependent on the specific process equipment and energy sources that are used, as well as the products made.
- The core near zero transformative pathway has the potential to reduce the subsector’s emissions to approximately 6 MMT CO2e by 2050, a 95% reduction compared with 2018. This reduction is largely driven by increased biomass use and improved energy efficiency of existing core unit processes, including drying and steam generation.
- The modeling was limited in that it lacked implementation of alternative pulping technologies, such as deep eutectic solvents and alternative fibers. Considering these alternative production routes can significantly change the impact of the interventions explored in the transformative pathways modeling.
- Electrification was considered for drying technologies and steam generation from the auxiliary boiler. Aggressive electrification of the auxiliary boiler (from 20% in the core near zero pathway to 50%) had minimal impact on emissions reduction. Steam generating heat pumps and more nascent electrification technologies were not considered as part of this but can presumably offer decarbonization potential.
- Broad adoption of the technologies in the core near zero transformative pathway minimizes the impact of recycling, imports, and demand reduction on the subsector’s decarbonization potential.
- While the estimated reductions reach near zero greenhouse gas emissions, biogenic emissions should be considered, especially due to increased biomass consumption proposed as the primary decarbonization intervention.
- This subsector has the opportunity to produce other value-added products through an integrated biorefinery approach. A cross-sectoral analysis is needed to evaluate the overall decarbonization impact of such an approach.
*Biogenic emissions are not considered in this work.
The plot below shows the necessary actions needed to reach near zero emissions for the core near zero pathway.
This graph shows U.S. annual greenhouse gas (GHG) emissions from 2018 through 2050 for the pulp and paper industry in a core near zero pathway. Labels refer to:
- Improve energy efficiency of key unit processes, including pulping, debarking, and drying.
- Electrify 20% of auxiliary boilers, clean grid by 2050.
- Fuel switching to biomass, e.g., wood chips and other solid biomass, and some renewable natural gas.
- Carbon capture of fossil-based emissions. Limited to 33% of remaining emissions in 2050.
Read More
Learn More and Get Involved
Learn more and read the latest news from the Industrial Efficiency and Decarbonization Office in the U.S. Department of Energy's Office of Energy Efficiency and Renewable Energy
Explore DOE's industrial technologies website to learn more about work across the department.
Get the latest news and opportunities for industrial efficiency and decarbonization!
Relevant News
-
Projects will drive industrial energy use and emissions down while increasing America’s manufacturing competitiveness in global marketsJanuary 8, 2025
-
Projects will drive industrial emissions down while increasing America’s manufacturing competitiveness in global markets.October 8, 2024
-
The Industrial Sustainability, Energy Efficiency, and Decarbonization (ISEED) Collaborative will provide assistance to partners across the manufacturing sector to develop and disseminate instructional curricula and training programs.September 16, 2024
-
On Sept. 3, 2024, the U.S. Department of Energy announced awards totaling $142 million for small businesses in 34 states. This investment includes $3.4 million for three projects funded by the Industrial Efficiency and Decarbonization Office.September 3, 2024
-
DOE announced approximately $15M to address adoption challenges preventing later stage commercialization, demonstration, and deployment of industrial decarbonization technologiesAugust 13, 2024
-
U.S. national laboratories can receive up to $9 million through an upcoming competitive lab call to develop and lead a Cement and Concrete Center of Excellence to accelerate the development and adoption of novel low-carbon cement and concrete technologiesJuly 19, 2024
-
In the latest round of cohorts in its Lab-Embedded Entrepreneurship Program (LEEP), 33 innovators will be embedded across four U.S. national laboratories to work with an extensive network of mentors and experts.July 10, 2024
-
The Framing the Future: Industrial Technologies Photo Contest will award $27,000 for the best photos of the innovative technologies, processes, and people that will help the United States achieve a decarbonized industrial sector.June 20, 2024
-
This request for information seeks to gain strategic and technical feedback on decarbonizing America’s industrial sector and positioning the United States as a leader in the global clean energy economyMay 14, 2024
-
DOE today announced its intent to issue multiple funding opportunity announcements totaling over $100 million for field demonstrations and other research to support better planning and operation of the electric grid.April 25, 2024