Researchers re-examined data from past experiments at Jefferson Lab to explore one way Lambda particles form.
November 30, 2023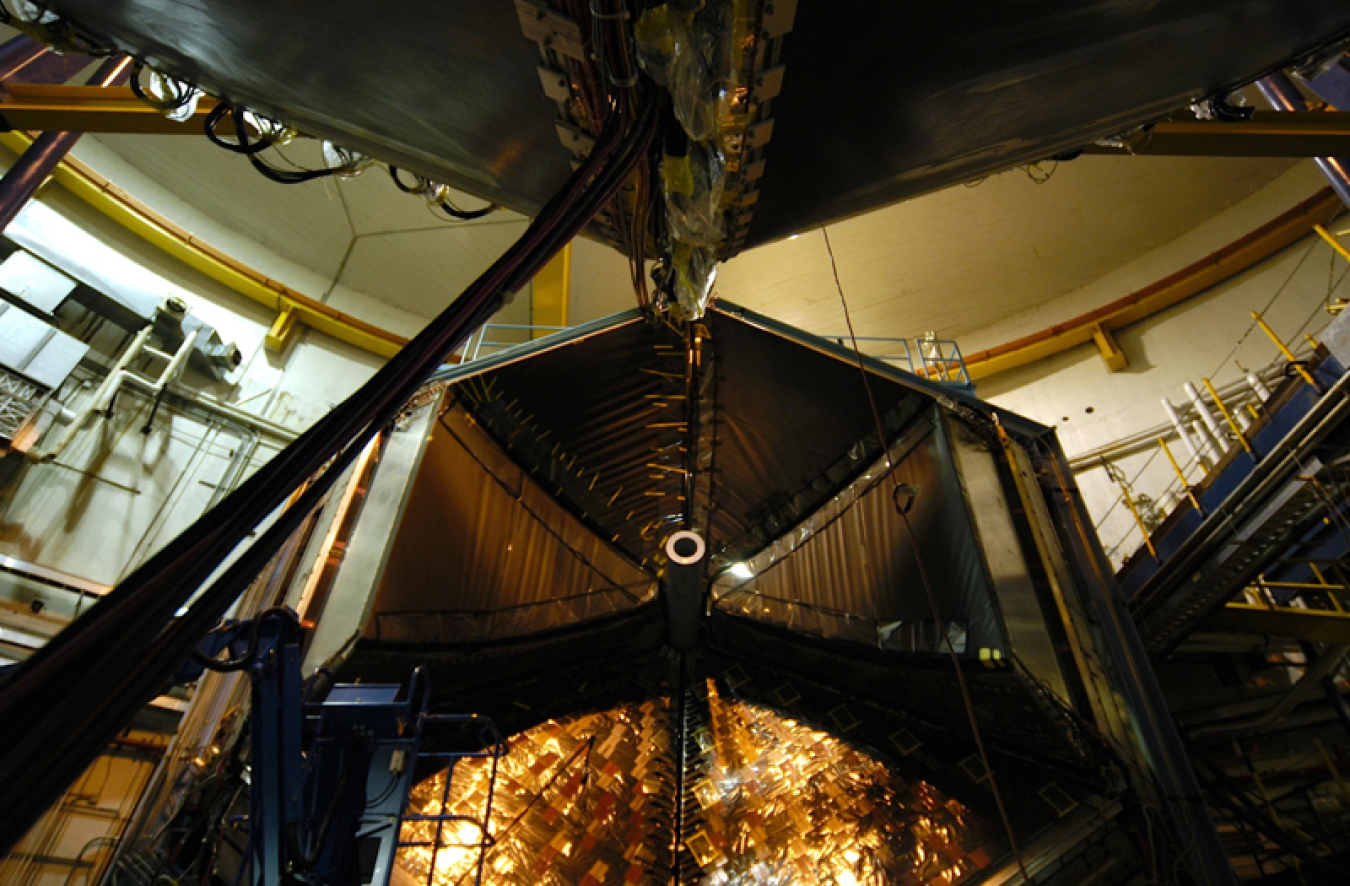
Sometimes, science is all about turning existing information on its head to get a new perspective. A “strange” perspective, in some cases.
As a PhD student working with nuclear physicists at the Department of Energy’s (DOE) Argonne National Laboratory, Lamiaa El Fassi used data from a 2004 experiment at the Continuous Electron Beam Accelerator Facility (CEBAF). A DOE Office of Science user facility, CEBAF is a huge particle accelerator at DOE’s Thomas Jefferson National Accelerator Facility (Jefferson Lab). CEBAF speeds up beams of electrons, which then smash into different targets. By looking at the electron beam bouncing off the target and the particles these collisions produce, nuclear scientists can better understand the insides of the nuclei that make up atoms.
Years later, El Fassi returned to that dataset with a new mission. As an assistant professor at Mississippi State University, she started sorting through the data to find evidence of how a specific process can produce “strange matter.”
Ordinary matter is made up of protons and neutrons, which are in turn made up of quarks and gluons. Strange matter is also made up of quarks and gluons. However, strange matter includes a different type of quark than ordinary matter. There are six types of quarks: up, down, charm, strange, top, and bottom. Protons and neutrons are made of combinations of up and down quarks. Strange matter can include up and down quarks, but always includes a strange quark as well. Strange matter can help us better understand the fundamental forces of the universe, particularly the strong force. That’s the force that holds quarks and gluons together.
Lambda particles can be a type of strange matter. That’s what El Fassi was searching for in the data. In particular, she wanted to see if there was some proof of a particular process in nuclear physics creating Lambda particles. This process is called semi-inclusive deep inelastic scattering. She wanted to understand if and how collisions of ordinary matter could result in strange matter.
In the experiment, CEBAF shot electrons at targets made of different materials, including carbon, iron, and lead. When an electron scattered off the target atom, the exchange particle hit the target’s nucleus. That impact caused a proton or neutron in the atom’s nucleus to break apart. When the proton or neutron broke apart, the electron’s probe had a chance to interact with a quark inside of that particle.
The electrons interacted with the target nucleus by exchanging a “virtual photon.” Regular photons are particles of light. Virtual photons don’t exist independently the way regular photons do. Instead, they’re an intermediate step when electrically charged particles (like protons or electrons) interact in a particular way. Virtual photons are responsible for the electromagnetic force – the force that holds together electrons and protons in atoms.
Once the virtual photon interacted with a quark, the quark started moving around as a free particle. Sometimes those quarks joined up with other existing quarks in the medium. In some of those cases, the quarks joined up in ways that became a Lambda particle.
But Lambda particles are extremely short lived. That’s one reason they’re so hard to study. In the experiment, they almost immediately broke down into two other particles.
To capture this super-quick process, El Fassi needed to do some detective work. The machine detected both the particles that resulted when the Lambda particle broke apart as well as the electron that bounced off the target. It’s like a pool table. Imagine a white cue ball (an electron) striking a cluster of balls (the proton). The cluster of balls breaks apart into new balls (the free quarks). The new balls roll around and sometimes form intermediate clusters (the Lamba particles). These clusters don’t last long and break apart into new balls altogether. Scientists can understand the intermediate clusters by studying the properties of the cue ball after it bounces off and the properties of the balls left at the end.
El Fassi and her team analyzed these data for years. It took so long because scientists had never before used this process to study Lambda particles in the current and target production regions. They had only used it on protons and lighter, more stable particles. But she pursued this method because it could lend new insights into how Lambda particles form.
After several years of research and a promotion to associate professor, El Fassi finally accomplished her goal! She made the first observation of Lambda particles produced by semi-inclusive deep inelastic scattering in the target production regions.
Surprisingly, the results showed that Lambda particles form in a way distinct from other particles. The other particles scientists had studied using this technique found that when an electron bounced off a nucleus, a virtual photon struck a single quark. That photon-quark interaction then formed the particle that scientists studied. But Lambda particles were strange – appropriate to their name of strange matter. Instead of the virtual photon interacting with a single quark, it was absorbed by a pair of quarks. That combined pair then would “find” a strange quark and form a Lambda particle.
Her initial finds are published, but El Fassi’s research is far from over. Her and her team’s results differ from what nuclear physics theory predicted should happen. Rather than being disappointing, having experimental results conflict with theory is exciting for scientists. It means there is something missing from the theory and there is more to discover.
El Fassi and other scientists are continuing to run experiments on CEBAF. Jefferson Lab recently upgraded it, so it’s even more powerful and versatile than before. In addition, the Electron-Ion Collider user facility is in the process of being built at DOE’s Brookhaven National Laboratory with support from Jefferson Lab. Once it’s done, it will provide new resources for scientists to study strange matter.
Nuclear physics is often pretty strange, especially when dealing with non-ordinary matter. Scientists like El Fassi and her team are finding new ways to explore these building blocks of the universe.
Shannon Brescher Shea
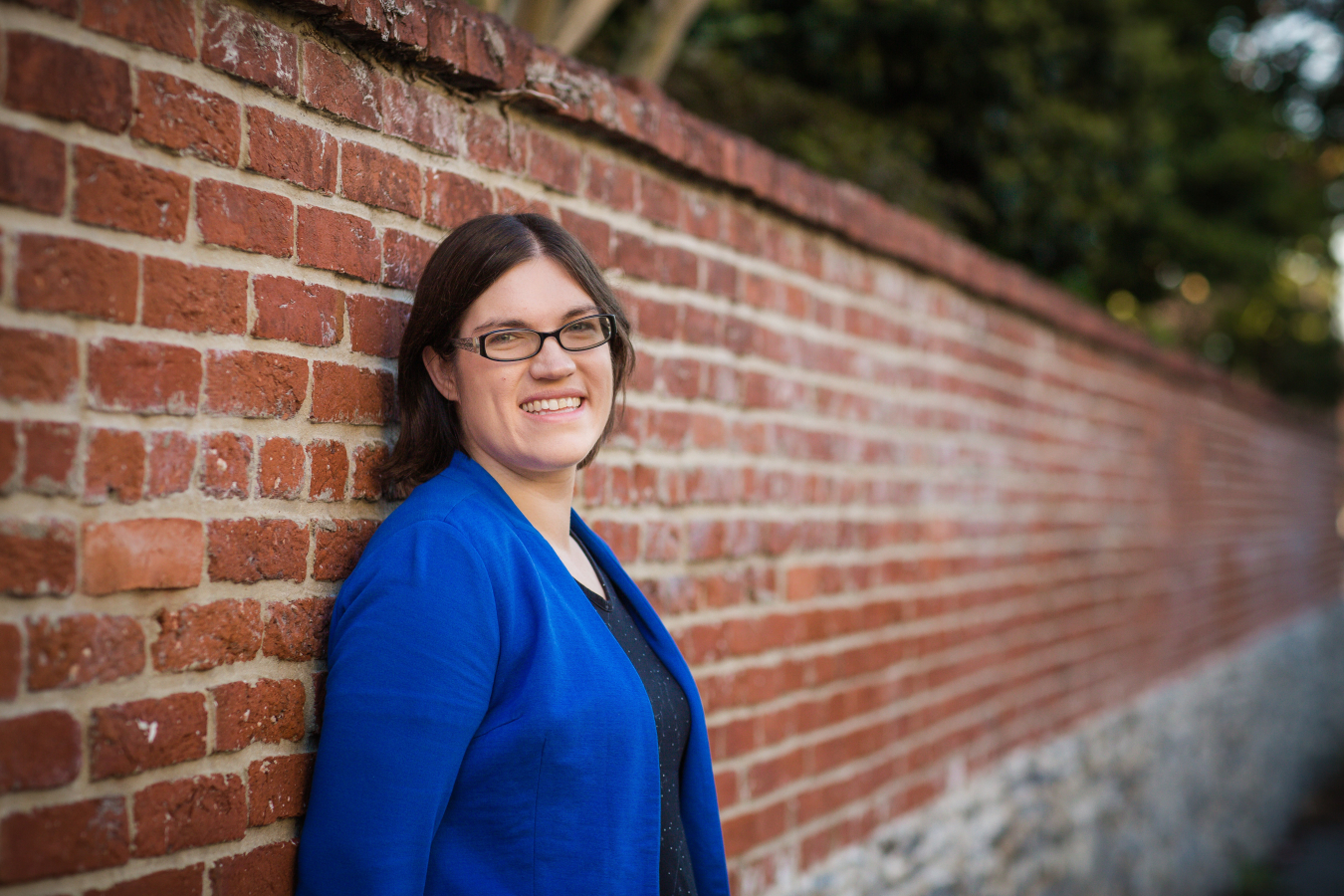
Shannon Brescher Shea ([email protected]) is the social media manager and senior writer/editor in the Office of Science’s Office of Communications and Public Affairs. She writes and curates content for the Office of Science’s Twitter and LinkedIn accounts as well as contributes to the Department of Energy’s overall social media accounts. In addition, she writes and edits feature stories covering the Office of Science’s discovery research and manages the Science Public Outreach Community (SPOC). Previously, she was a communications specialist in the Vehicle Technologies Office in the Office of Energy Efficiency and Renewable Energy. She began at the Energy Department in 2008 as a Presidential Management Fellow. In her free time, she enjoys bicycling, gardening, writing, volunteering, and parenting two awesome kids.